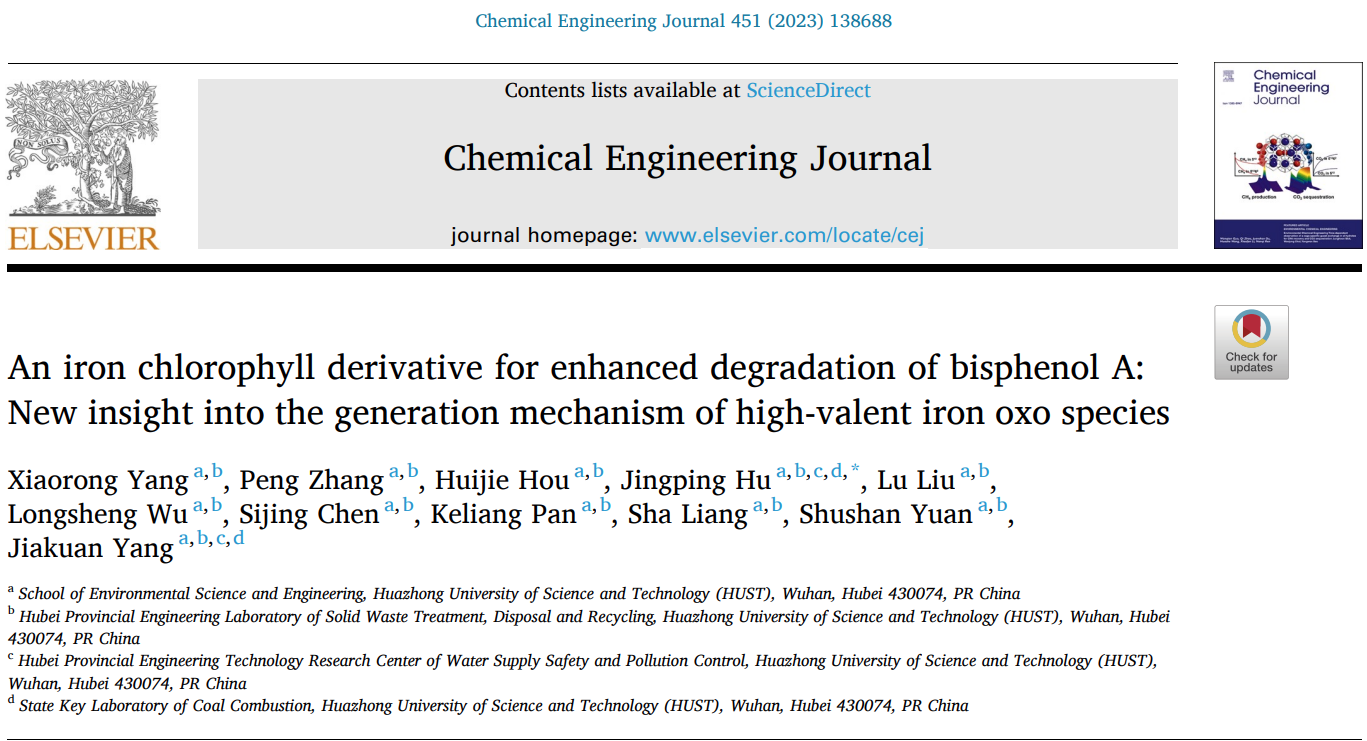
第一作者:杨小容、张鹏
通讯作者:胡敬平
通讯单位:华中科技大学环境科学与工程学院
论文DOI: 10.1016/j.cej.2022.138688
论文全文链接:https://doi.org/10.1016/j.cej.2022.138688
图文摘要
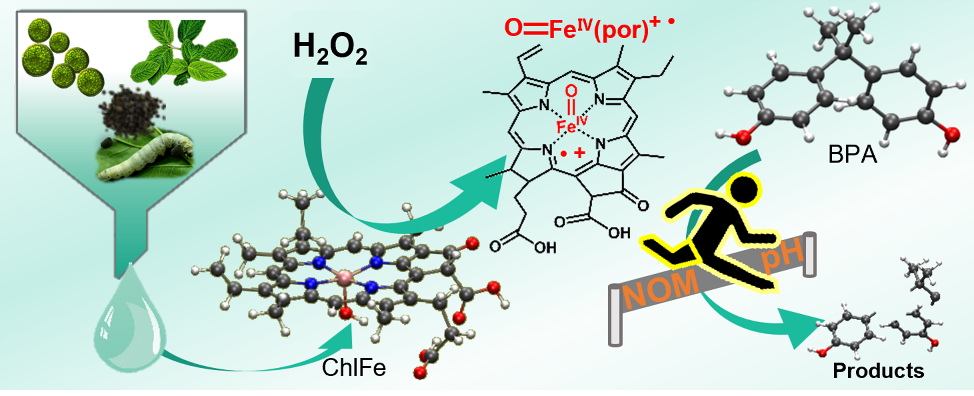
成果简介
近日,华中科技大学环境学院FunMat课题组胡敬平教授团队在Chemical Engineering Journal上发表了题为“An iron chlorophyll derivative for enhanced degradation of bisphenol A: New insight into the generation mechanism of high-valent iron oxo species” (Chemical Engineering Journal, 451 (2023) 138688. https://doi.org/10.1016/j.cej.2022.138688)的研究论文。该研究通过从蚕砂中提取出叶绿素,并对其进行皂化和置铁反应制备了铁叶绿素衍生物,将其作为催化剂结合过氧化氢用于目标污染物的去除。研究结果表明相比同等投料比下的传统Fenton体系,铁叶绿素体系降解双酚A的速率更快,在pH为4~12的范围内都可以达到近100%的去除率,且抗水体共存组分的干扰。其中对双酚A的去除起贡献的活性物质是高价铁氧物种,研究通过实验和理论计算结合的方式揭示了一种非典型的O−O键均裂产生高价铁氧卟啉π阳离子自由基(O=FeIV(Por)+•)的活化机制。
引言
依赖氧中心自由基为主要活性物种的高级氧化技术被广泛应用于废水治理中,但由于氧中心自由基的活性高选择性低容易被水体共存组分淬灭的问题,使这类高级氧化技术在微污染物治理的应用具有局限性。比起氧中心自由基,高价铁物种的活性相对较低且对底物具有独特的氧化行为,即使在高浓度的无机离子或有机质存在的情况,仍能高效的选择性降解目标污染物。以血红素酶为基础的生命活动过程中能产生高价铁氧卟啉中间体代谢内源性或外源性物质,把作用机理从自然界转移到实验室甚至工业化水平的仿生研究一直备受青睐。仿生卟啉的大量合成促进了高价铁氧卟啉中间体的产生机制研究。其中Traylor团队认为过氧化物在金属卟啉的催化下其O−O键异裂产生高价铁氧卟啉π阳离子自由基(O=FeIV(Por)+•,化合物I),而Bruice团队认为O−O键均裂形成高价铁氧卟啉(O=FeIV(Por),化合物II)和氧中心自由基(•OR),Nam团队则认为O−O键既可以均裂也可以异裂,这取决于金属卟啉与过氧化物本身的电子特性。然而,大多数人工合成卟啉都是meso位取代的卟啉,对高价铁氧卟啉中间体的产生机制也都是基于对meso位取代的铁卟啉进行探索的。实际上,自然界中的金属卟啉多以β位取代的形式存在。因此本研究通过从蚕砂中提取叶绿素进行皂化置铁制备了铁叶绿素衍生物,通过实验与理论计算结合的研究方法探索了其活化过氧化氢产生高价铁氧物种的机制。
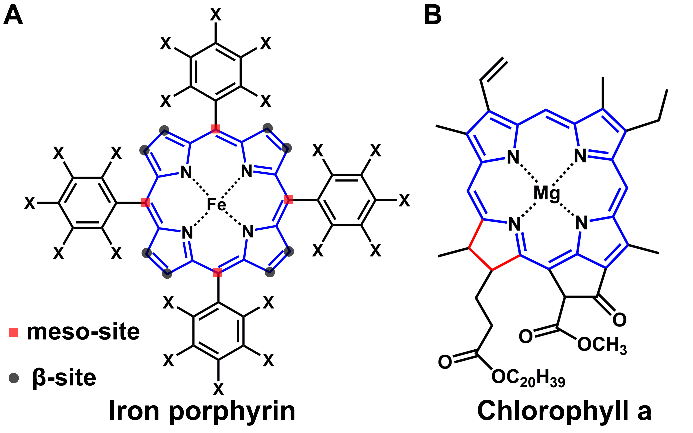
Fig. 1. (A) Structure of iron porphyrin complexes. (B) Structure of chlorophyll a.
图文导读
材料的表征
通过SEM/EDS对材料的形貌进行表征,通过原位红外、紫外光谱以及质谱对材料的结构进行了系列表征,确定了制备材料的分子结构。
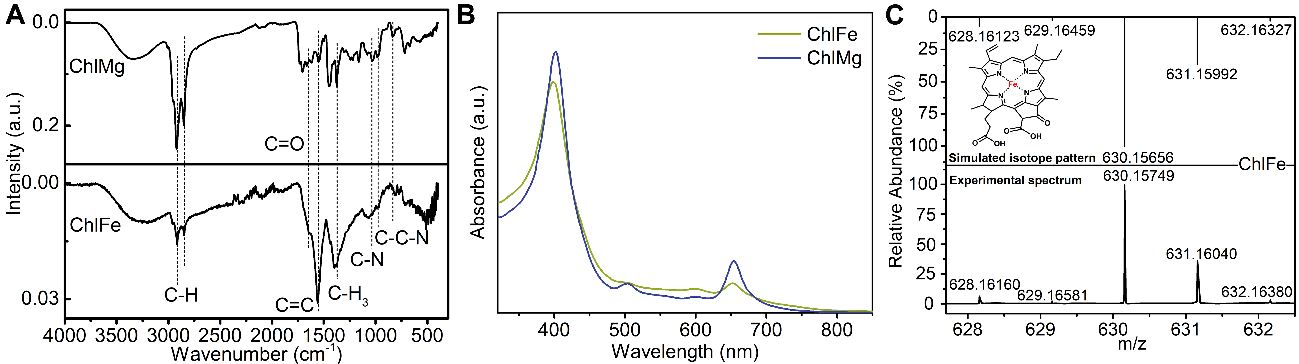
Fig. 2. Characterization of ChlFe. (A) FT-IR spectra and (B) UV–vis spectra of ChlFe and ChlMg. (C) Acquired mass spectrum in ESI-MS analysis of ChlFe and isotope pattern simulated for the molecular structure in the inset.
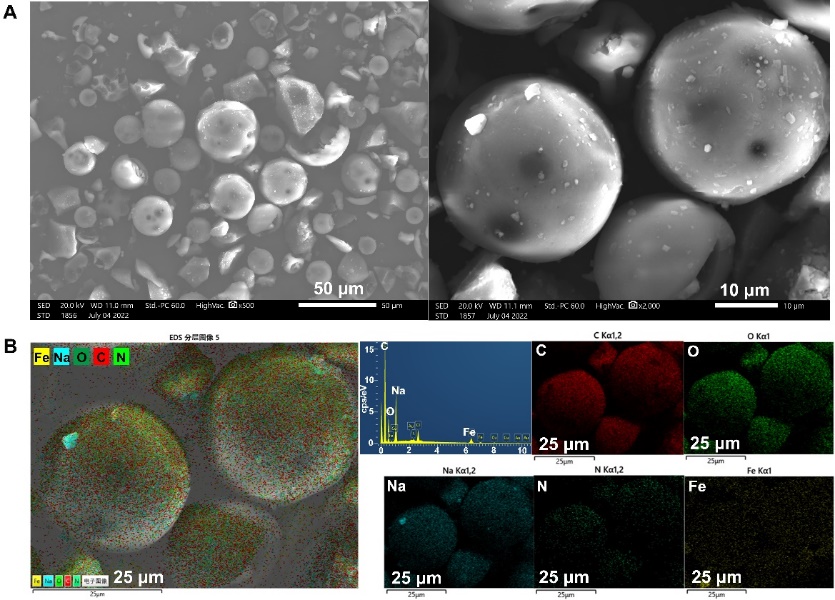
Fig. 3. (A) SEM images and (B) EDS mapping of ChlFe.
去污性能
以双酚A为目标污染物对投加的催化剂和氧化剂的浓度进行优化。通过反应体系的对比体现铁叶绿素衍生物(ChlFe)/H2O2体系优异的去污性能,并研究了该体系的pH适应性,抗离子干扰性,以及循环稳定性。
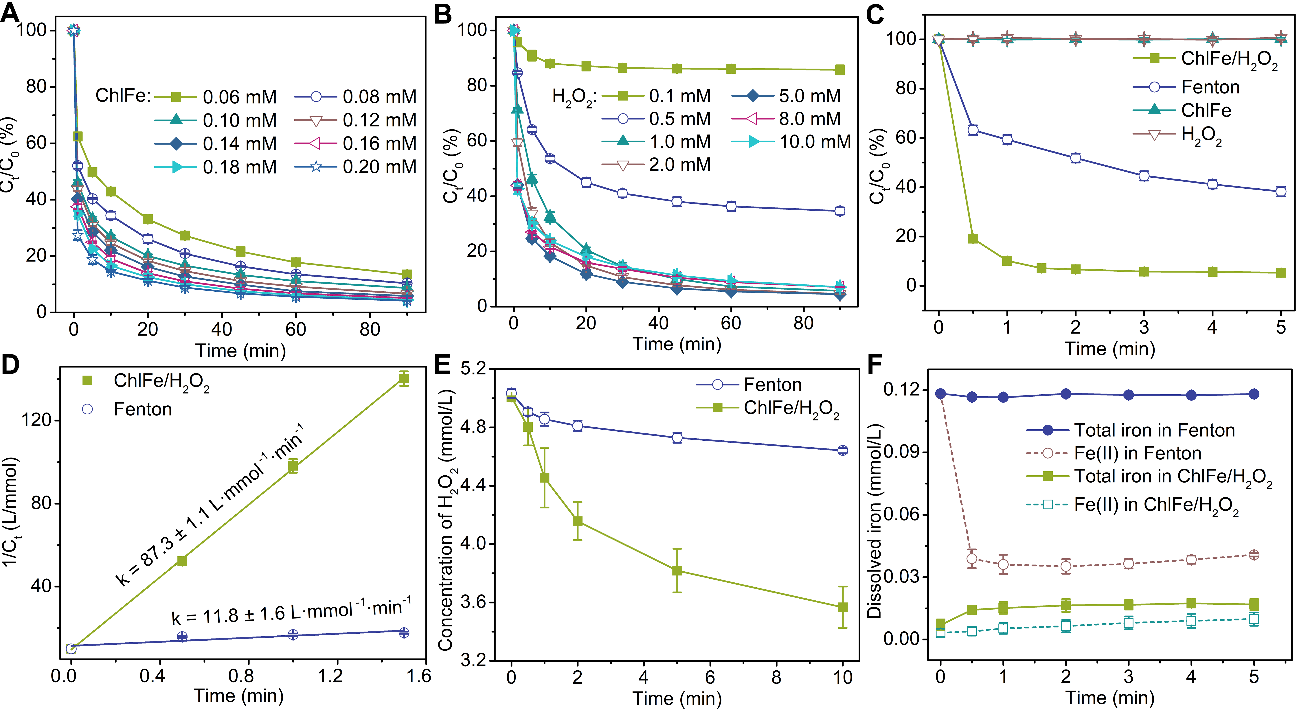
Fig. 4. Degradation of BPA with different concentrations of (A) ChlFe and (B) H2O2 at initial pH = 7.0. (C) Degradation of BPA in different catalytic systems. (D) Pseudo second order rate constants for the degradation of BPA in the ChlFe/H2O2 system and Fenton system. (E) Variation in hydrogen peroxide concentration in the different systems. (F) The release of ferrous ions and total iron ions during BPA degradation in different catalytic systems. Initial experimental conditions: Fe(Ⅱ) = 0.12 mM in Fenton system at pH = 2.7; ChlFe = 0.12 mM in ChlFe/H2O2 system at pH = 9, H2O2 = 5.0 mM, BPA = 0.10 mM, HCO3− = 100 mM, Cl− = 100 mM, SO42− = 100 mM, and HA = 20 mg·L−1.
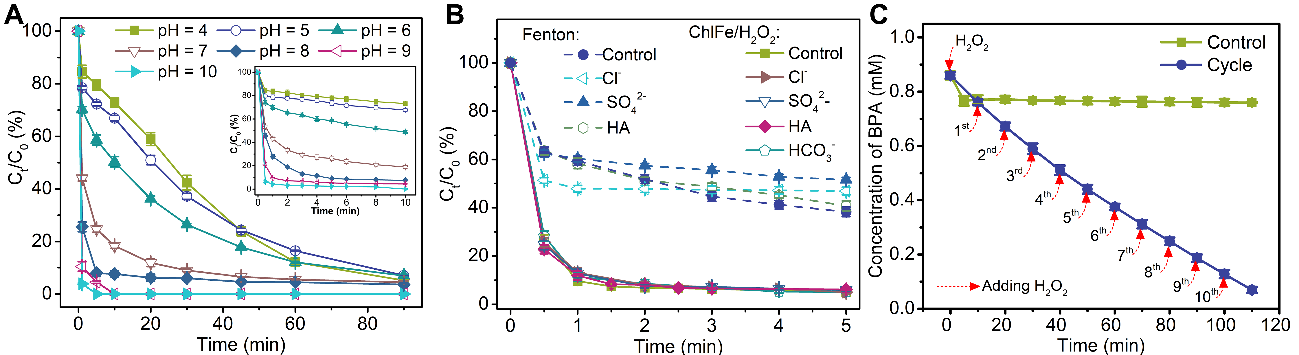
Fig. 5. (A) Degradation performance in the ChlFe/H2O2/BPA system within 90 min at different initial pH values of 4.0 - 10.0, with an enlarged view in the inset within the first 10 min. Curves of pH 11 and 12 are omitted for clarity. (B) The influence of NOM and the inorganic anions Cl−, SO42−, and HCO3− on the degradation of BPA in the Fenton system and ChlFe/H2O2 system. Initial experimental conditions: Fe(Ⅱ) = 0.12 mM in Fenton system at pH = 2.7; ChlFe = 0.12 mM in ChlFe/H2O2 system at pH = 9, H2O2 = 5.0 mM, BPA = 0.10 mM, HCO3− = 100 mM, Cl− = 100 mM, SO42− = 100 mM, and HA = 20 mg·L−1. (C) Cycle tests showing BPA degradation in ChlFe/H2O2 system. Initial experimental conditions: ChlFe = 0.06 mM, BPA = 0.86 mM, H2O2 = 2.5 mM for each dose.
活性物种探索
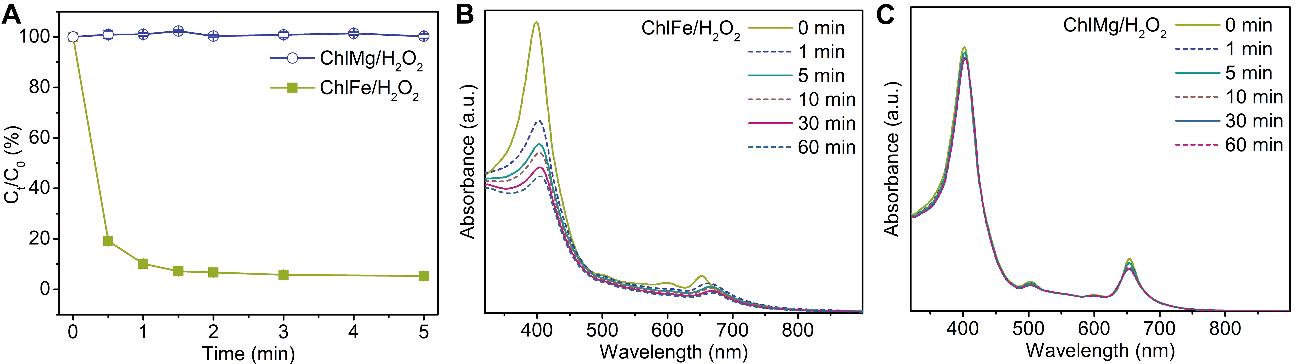
Fig. 6. (A) BPA degradation profile with ChlFe and ChlMg used as catalysts. UV–Vis spectra of (B) ChlFe and (C) ChlMg with H2O2 in aqueous solution. Initial experimental conditions: Fe (Ⅱ) = 0.12 mM in Fenton system at pH = 2.7, ChlFe = 0.12 mM in ChlFe/H2O2 system at pH = 9, H2O2 = 5.0 mM, ChlMg = 0.12 mM, BPA = 0.10 mM.
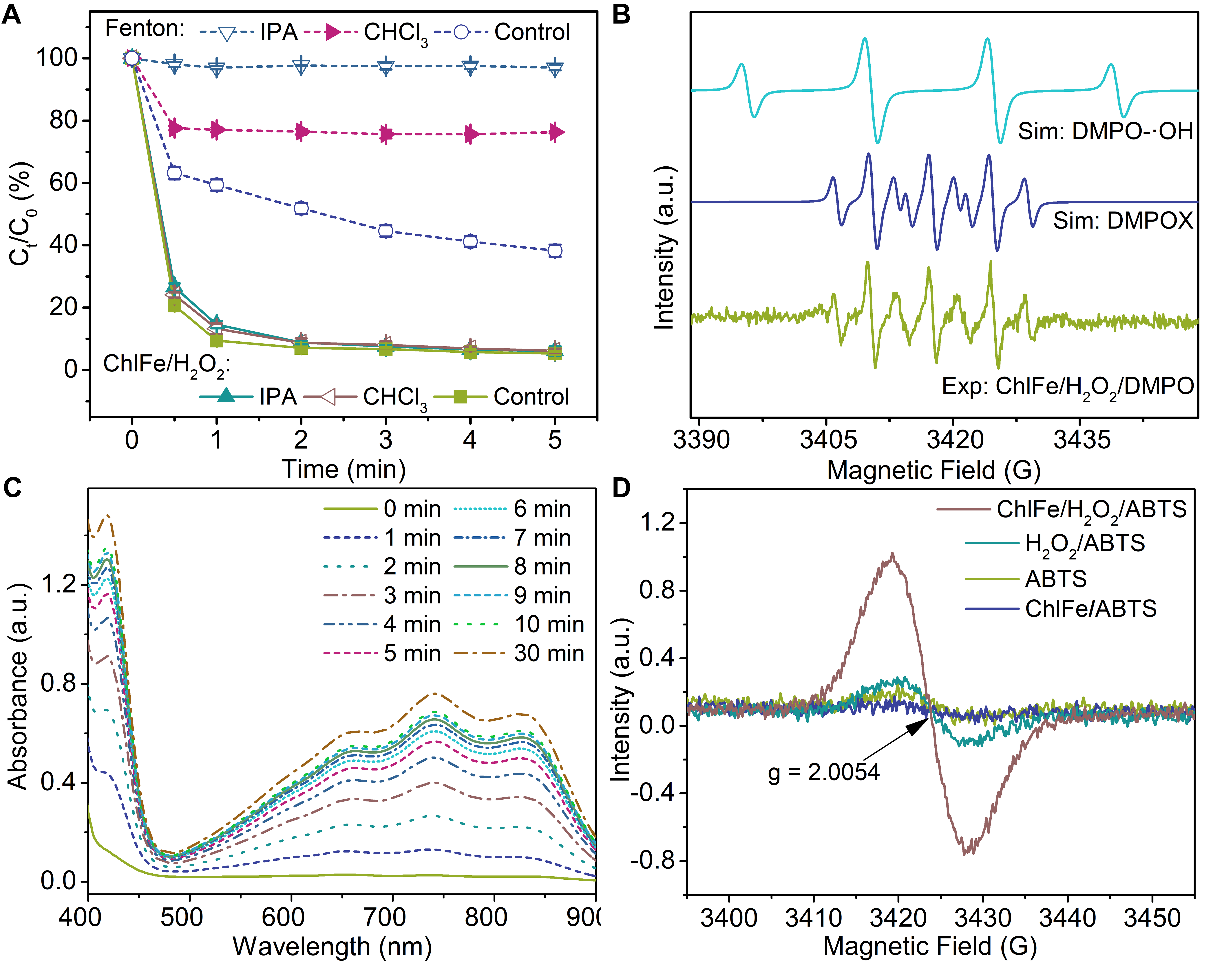
Fig. 7. (A) Degradation of BPA in radical quenching experiments with the different radical scavengers isopropanol (IPA) and chloroform (CHCl3). (B) EPR spectra of experiments (Exp) and simulation (Sim) in the ChlFe/H2O2 system when DMPO was used as the trapping reagent. (C) UV–Vis spectra of the mixture in the ChlFe/H2O2/ABTS system at pH = 9. (D) EPR spectra of ChlFe and H2O2 with ABTS introduced as a trapping reagent at pH = 9.0 and room temperature. Initial experimental conditions: Fe (Ⅱ) = 0.12 mM in Fenton system at pH = 2.7, ChlFe = 0.12 mM in ChlFe/H2O2 system at pH = 9, H2O2 = 5.0 mM, ChlMg = 0.12 mM, BPA = 0.10 mM, IPA = 100 mM, and CHCl3 = 100 mM, ABTS = 35 mM.
通过对比叶绿素/H2O2体系, ChlFe/H2O2体系的反应位点为中心铁与过氧化氢的反应。但通过与传统Fenton体系的对比,ChlFe/H2O2体系降解双酚A的主要活性物种不是类似传统Fenton体系中的氧中心自由基,通过探针实验、质谱检测以及穆斯堡尔表征证明了ChlFe/H2O2体系中的活性物种为高价铁氧卟啉。
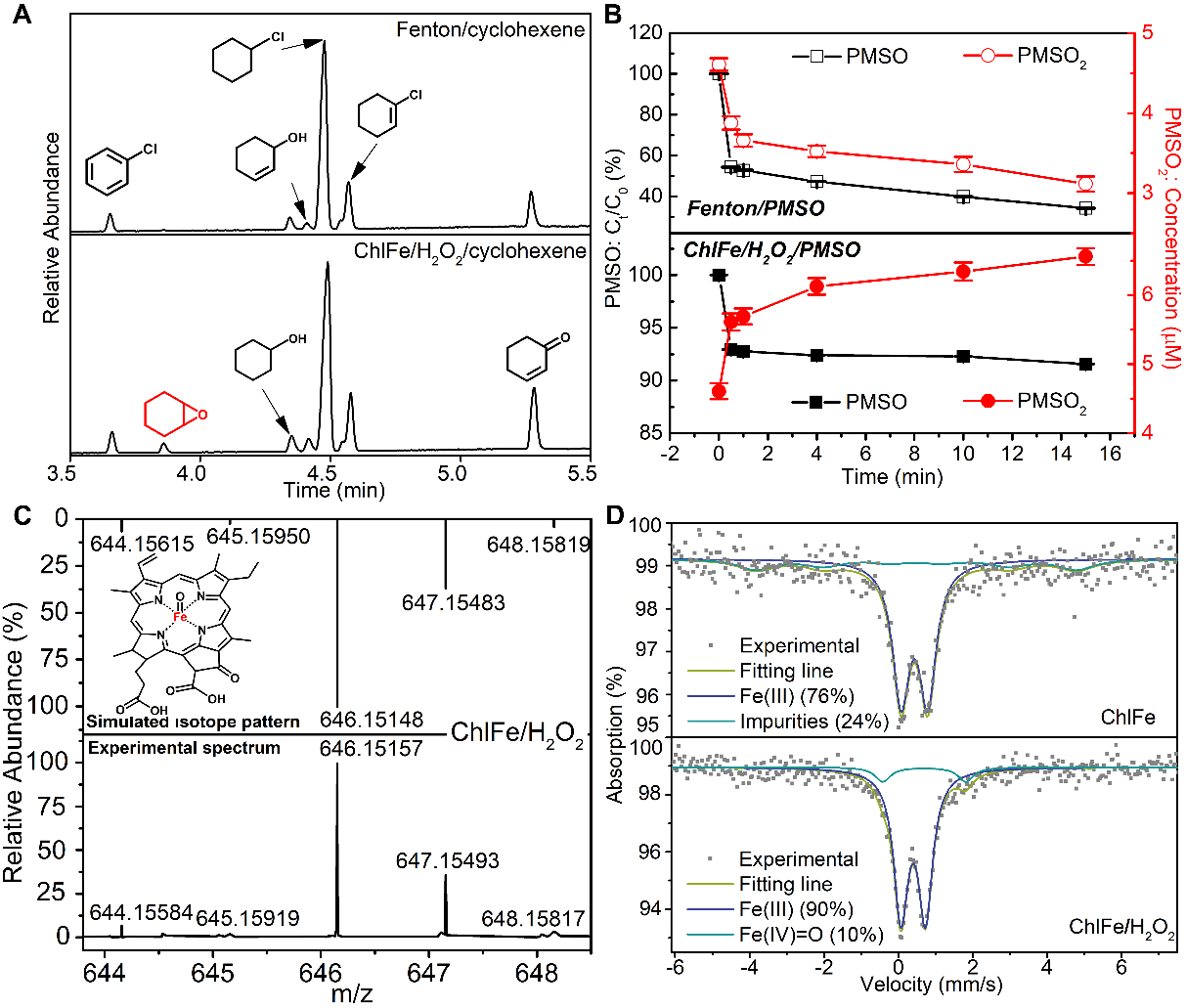
Fig. 8. (A) GC–MS chromatograms obtained after 30 min of the epoxidation reactions in Fenton/cyclohexene system at initial pH = 2.7 and ChlFe/H2O2/cyclohexene system at initial pH = 9.0, room temperature. (B) The variation of percentage oxidation of PMSO and the concentration of PMSO2 in Fenton system at initial pH = 2.7 and ChlFe/H2O2 system at initial pH = 9.0. (C) ESI-MS spectrum of the reaction mixture of ChlFe and H2O2 in methanol at 30 sec and isotope pattern simulated for molecular structure in figure inset. (D) Zero-field Mössbauer spectra of ChlFe and a mixture of ChlFe with H2O2 at 77 K. Experimental conditions: ChlFe = 0.12 mM, Fe(II) = 0.12 mM, PMSO = 200 μM, H2O2 = 5.0 mM.
活化机制
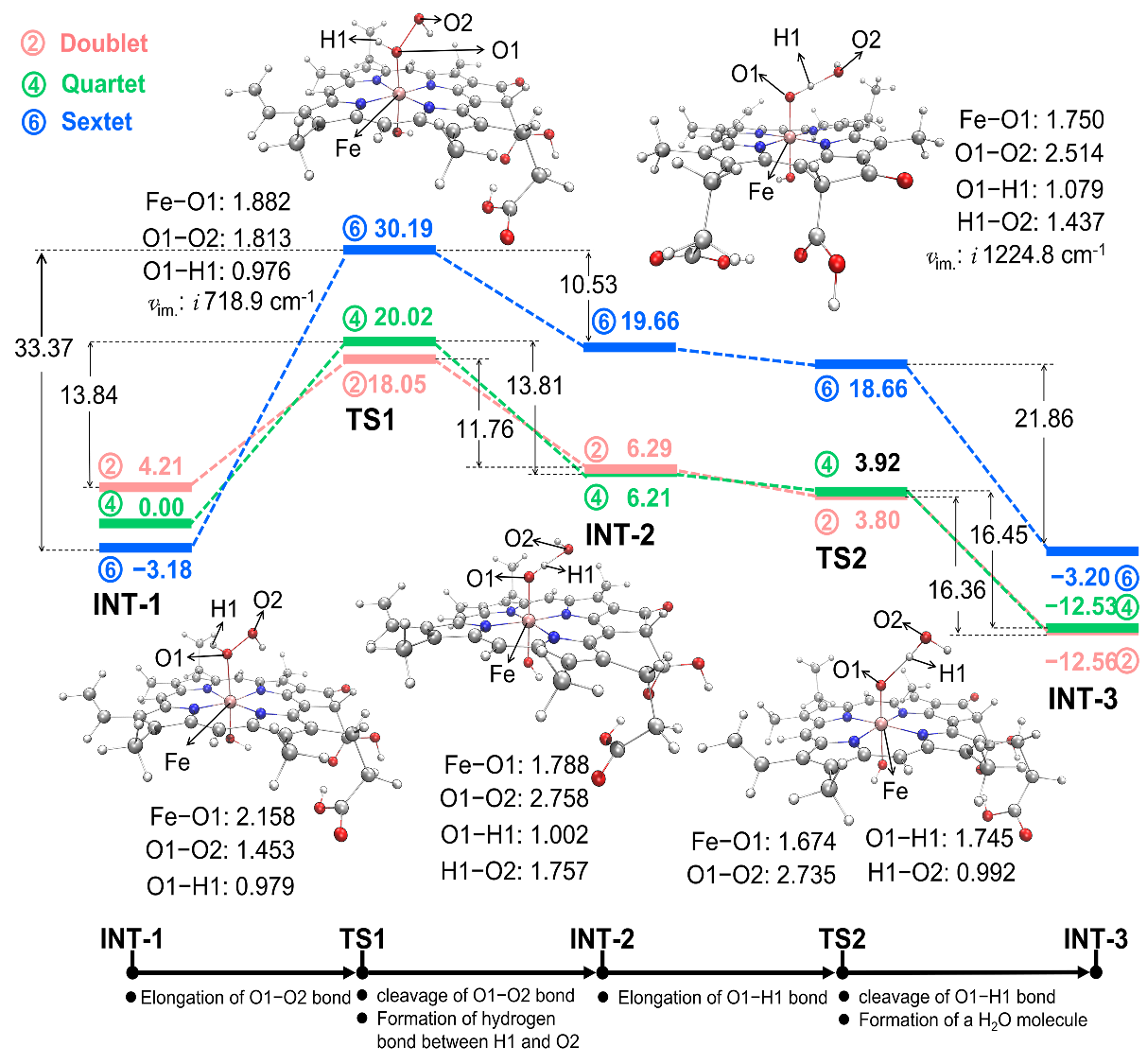
Fig. 9. Energy schemes for the reaction between ChlFe and H2O2, along with the optimized geometries and bond lengths (Å) of the key intermediates in the doublet state. Energies (kcal/mol) are relative to INT-1 in quartets. The transition states (TS1 and TS2) are confirmed by the imaginary frequencies.
通过理论计算研究了活性物种高价铁氧卟啉的产生机制,即过氧化氢首先在ChlFe的轴向形成配位,接着其O−O键逐渐拉伸断裂,通过对该复合物各片段的自旋布居、氧化态以及电子密度进行分析,总结出一种铁卟啉催化过氧化物的非典型活化机制。即,由于卟啉环上β位的丙酸基团的吸电子效应,O−O键首先发生均裂产生高价铁羟基卟啉(HO−FeIV(Por))和•OH,卟啉环通过大π键传递一个电子到•OH,同时过氧化氢的另一个H以质子形式转移到•OH,即电子耦合质子转移的方式最终形成高价铁氧卟啉阳离子自由基O=FeIV(Por)+•。
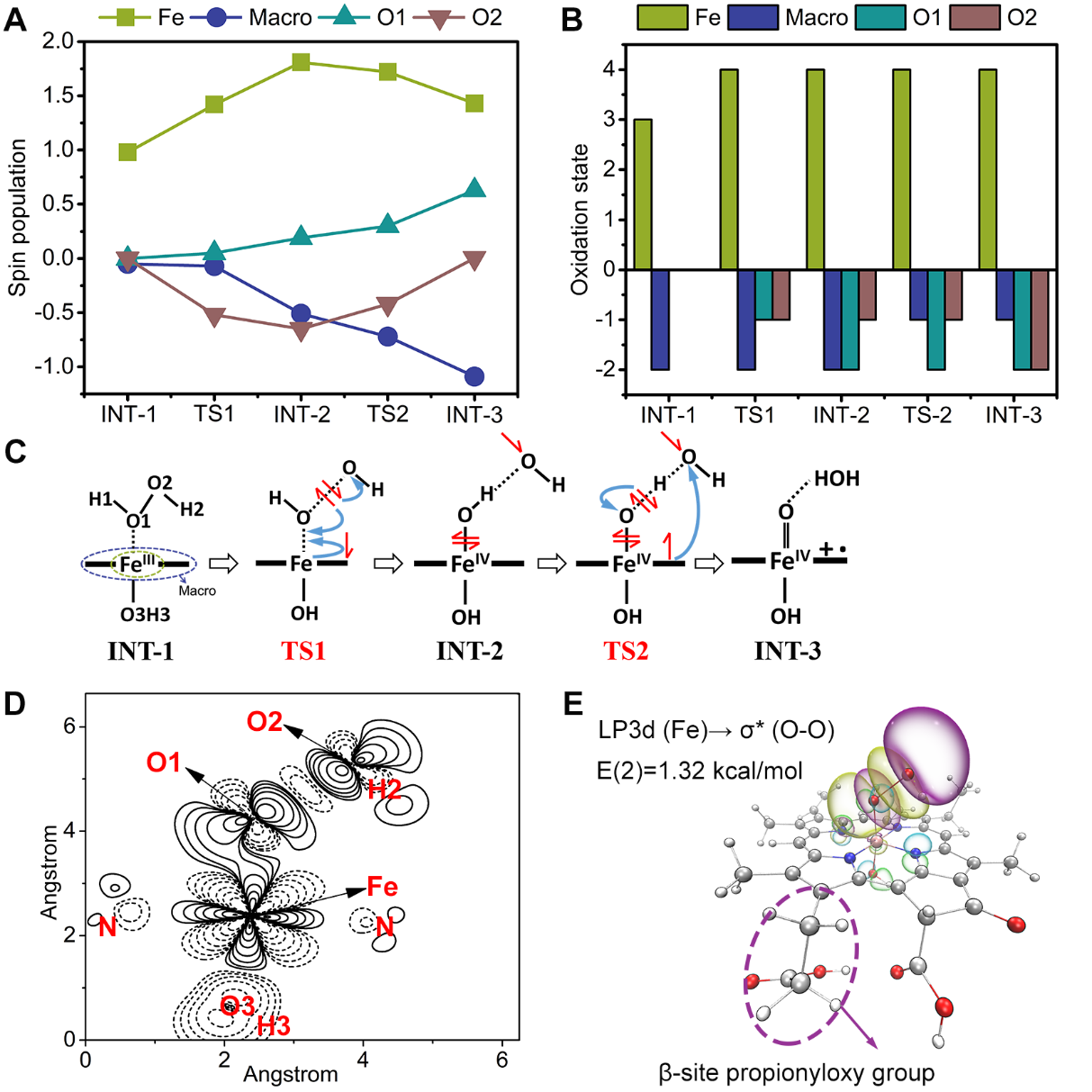
Fig. 10. (A) Spin population and (B) oxidation state of the atomic groups of intermediates: O2, Fe, O1 and Macro, where Macro represents chlorophyll derivate ligand without Fe. (C) The activation pathway of H2O2 by ChlFe. (D) The difference in electron density of TS1 after substitution with the propionyloxy group at the β site of the chlorin ring, with TS1 divided into two parts: the propionyloxy and the rest. (E) Hyperconjugation interaction of iron 3d orbitals with antibonding O−O of hydroperoxide of the transition state TS1 from natural bond orbital analysis, with an isovalue of ±0.005.
双酚A降解路径的确定
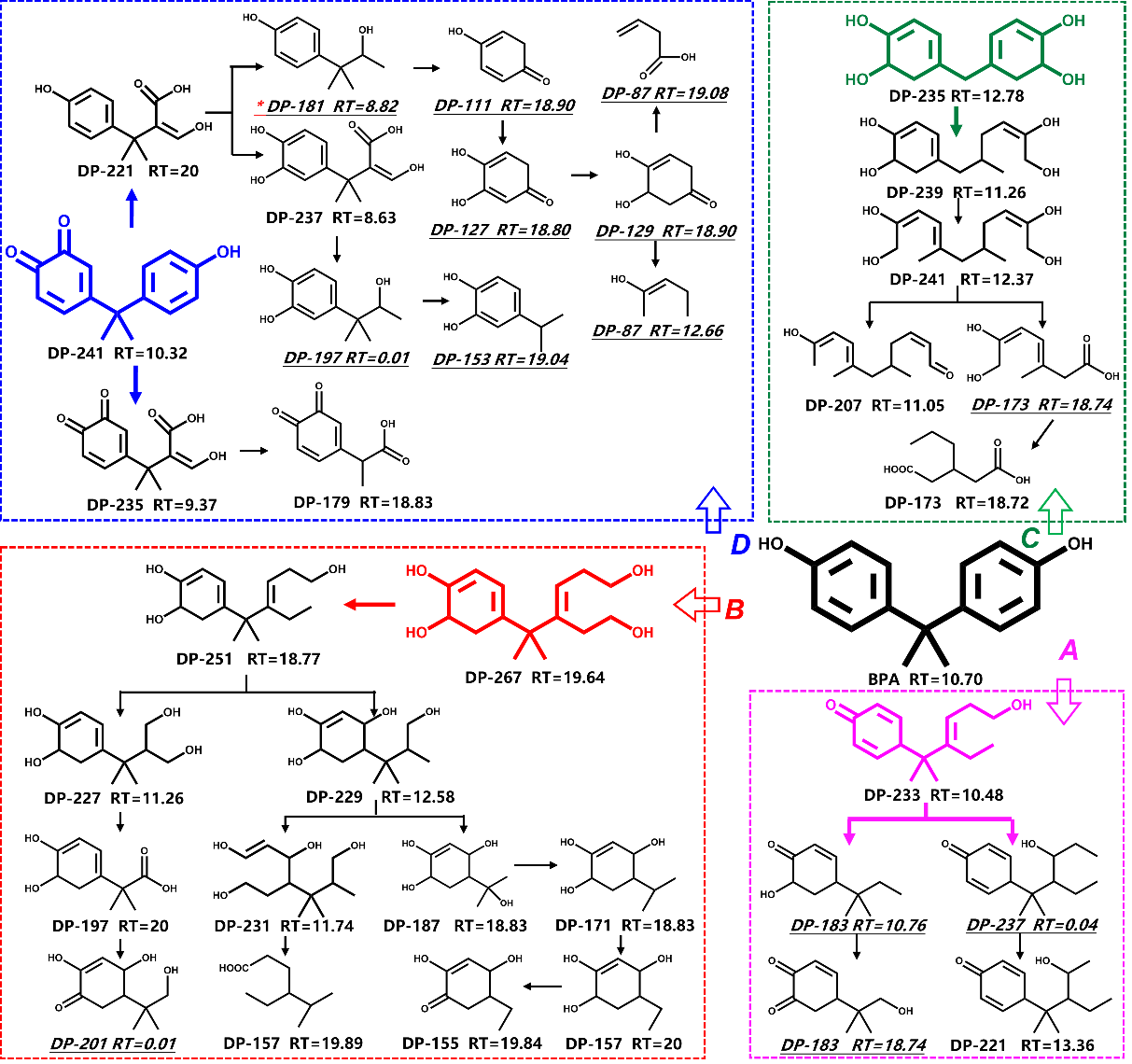
Fig. 11. Proposed degradation pathways of BPA in the ChlFe/H2O2 process. *The products in italics with underlines were acquired in positive ions mode, while others in negative ions mode.
结论
该研究从蚕砂中提取叶绿素并通过皂化置铁制备了铁叶绿素衍生物,在过氧化氢的驱动下,体系中产生了高价铁氧卟啉阳离子自由基活性物种,可以快速高效地去除双酚A,适用pH范围广且抗共存离子及有机质的干扰。通过密度泛函计算揭示了一种受卟啉环上β位的丙酸基团修饰的非典型活化机制,过氧化氢在铁叶绿素衍生物的活化下,O−O键首先发生均裂产生高价铁羟基卟啉(HO−FeIV(Por))和•OH,卟啉环通过大π键传递一个电子到•OH,同时过氧化氢的另一个H以质子形式转移到•OH,即电子耦合质子转移的方式最终形成高价铁氧卟啉阳离子自由基O=FeIV(Por)+•。
作者简介
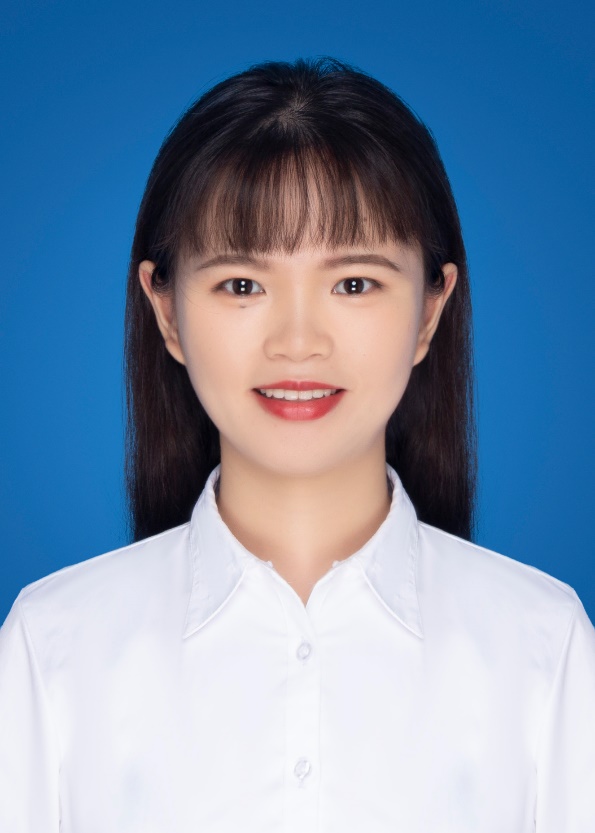
杨小容,博士研究生,华中科技大学环境学院,研究方向为高级氧化技术的探索及其应用。
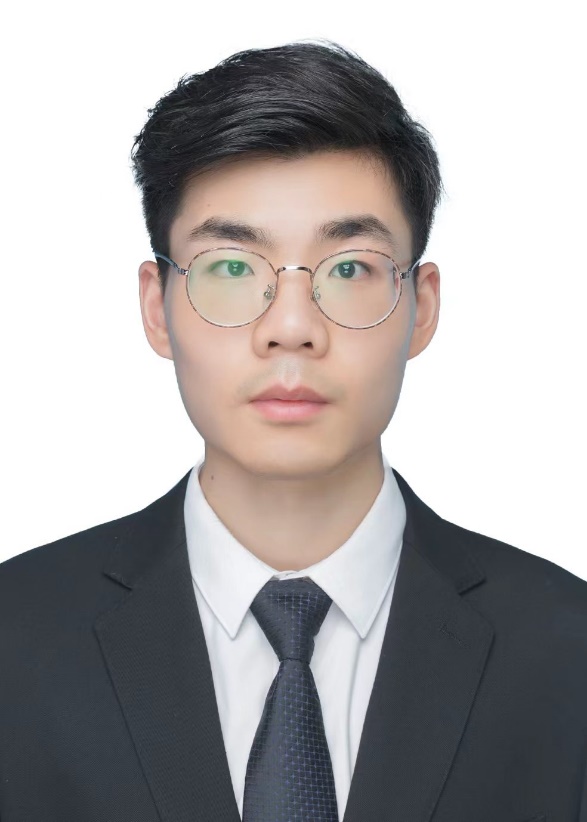
张鹏,硕士毕业于华中科技大学环境学院。研究方向为高级氧化技术在废水治理方面的应用。在Chemosphere上发表综述一篇(Chemosphere 219 (2019) 617-635,Recent advances in metalloporphyrins for environmental and energy applications),授权发明专利一项(ZL201810274621.7,一种过渡金属叶绿素衍生物类芬顿反应试剂及其应用)。
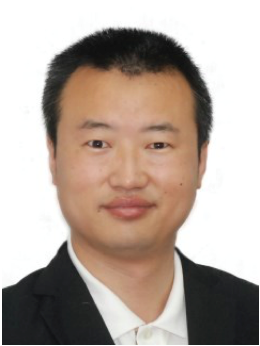
胡敬平,教授、博导,华科大环境学院实验中心主任。2008年博士毕业于英国牛津大学,博士毕业后先后在英国诺 丁汉大学和牛津大学从事博士后研究工作,2011年当选牛津大学拉姆齐研究学者(Ramsay Fellow)。聚焦环境科学与工程领域,开展固废资源化、环境仿生催化、环境大数据的研究。主持英国The Ramsay Memorial Fellowships Trust项目、国家自然科学基金面上项目与青年项目、湖北省自然科学重点基金、国家重点研发计划“固废专项”的课题,承担科技部“青年973”项目和国家自然科学基金创新群体项目,在Adv. Mat.、Angew. Chem.、Adv. Func. Mat.等期刊发表论文170余篇,其中近五年第一作者及通讯作者论文32篇;单篇最高他引170余次,总他引超过4670次,H因子38(Jingping Hu (0000-0001-9984-6636) (orcid.org)),授权发明专利10余项(含3项国际发明专利)、软件著作权2项。担任国际期刊“Energy & Environmental Materials”副主编、巴塞尔公约亚太区域中心化学品和废物环境管理智库专家、湖北省资源综合利用协会专家委员会委员。
文献链接
Xiaorong Yang, Peng Zhang, Huijie Hou, Jingping Hu*, Lu Liu, Longsheng Wu, Sijing Chen, Keliang Pan, Sha Liang, Shushan Yuan, Jiakuan Yang, An iron chlorophyll derivative for enhanced degradation of bisphenol A: New insight into the generation mechanism of high-valent iron oxo species, Chemical Engineering Journal, 451 (2023) 138688. https://doi.org/10.1016/j.cej.2022.138688